Presented By: Biomedical Engineering
PhD Defense: Sabrina Lynch
Non-Newtonian Stabilized Mass Transport Model: Applications to Thrombosis Research in Cardiovascular Hemodynamics
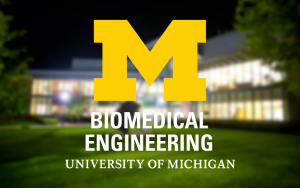
NOTICE: This event will be held via Zoom. The link will be provided below.
Zoom link: https://umich-health.zoom.us/j/94668154127
Thrombosis is a process whereby a blood clot forms in situ within a vessel and impedes flow. Although necessary to maintain hemostasis, the human thrombotic system often becomes unstable leading to scenarios of thrombosis and subsequent diseases such as myocardial infarction, stroke, pulmonary embolism, and deep vein thrombosis. Computational modeling is a powerful tool to understand the complexity of thrombosis initiation and provides both temporal and spatial resolution that cannot be obtained in vivo. The goal of this investigation is to develop a computational model of thrombosis initiation in patient-specific models that includes both a complex description of the hemodynamics and biochemistry of thrombin formation. We argue that the complex hemodynamics occurring in vivo significantly alter the initiation and progression of thrombosis.
While blood viscosity is known to exhibit nonlinear behavior, a Newtonian assumption is often employed in computational analyses. This assumption is valid in healthy arteries where shear rates are high and recirculation is low. However, in pathological geometries, such as aneurysms, and venous geometries, this assumption fails, and nonlinear viscous effects become exceedingly important. Previous computational models of thrombosis have investigated coagulation through chemistry based formulations focusing on protein dynamics but have generally excluded complex 3D hemodynamics.
A computational framework was developed to investigate the interplay between 3D hemodynamics and the biochemical reactions involved in thrombosis initiation in patient-specific models under transient flow. The salient features of the framework are: i) nonlinear rheological models of blood flow; ii) a stabilized numerical framework for scalar mass transport; and iii) a computational interface for nonlinear scalar models of protein dynamics that can be easily customized to include an arbitrary number of species and protein interactions.
We implemented and verified nonlinear rheological models of viscosity into CRIMSON and investigated the effects of non-Newtonian viscosity on both hemodynamic and transport metrics in an arterial and venous patient-specific model. Results demonstrated the importance of considering accurate rheological models.
A stabilized finite element (FE) framework was developed to solve scalar mass transport problems in CRIMSON. Simulation of cardiovascular scalar mass transport problems offers significant numerical challenges such as highly advective flows and flow reversal at outlet boundaries. Furthermore, little attention has been given to the identification of appropriate outflow boundary conditions that preserve the accuracy of the solution. These issues were resolved by developing a stabilized FE framework that incorporates backflow stabilization for Neumann outlet boundaries; a consistent flux boundary condition that minimally disturbs the local physics of the problem; and front-capturing stabilization to regularize solutions in high Pe number flows. The efficacy of these formulations was investigated for both idealized and patient-specific geometries.
Next, a flexible arbitrary reaction-advection-diffusion (ARAD) interface was implemented that enables prototyping nonlinear biochemical models of thrombin generation. After verifying the ARAD interface, the performance was compared against the original hardcoded FORTRAN implementation for speed and accuracy using a 4-scalar nonlinear reaction model of thrombosis. Three different biochemical models of thrombin generation were investigated in idealized geometries. Finally, we implemented the 18 scalar model in both idealized and patient-specific geometries to determine the effects of complex 3D hemodynamics on thrombin generation.
The computational framework for thrombosis initiation presented in this work has three key features: i) non-Newtonian hemodynamics; ii) a stabilized numerical framework for scalar RAD problems; and iii) a method to rapidly prototype custom reaction models using Python with negligible associated computational expense.
Chair: Prof. Alberto C. Figueroa
Zoom link: https://umich-health.zoom.us/j/94668154127
Thrombosis is a process whereby a blood clot forms in situ within a vessel and impedes flow. Although necessary to maintain hemostasis, the human thrombotic system often becomes unstable leading to scenarios of thrombosis and subsequent diseases such as myocardial infarction, stroke, pulmonary embolism, and deep vein thrombosis. Computational modeling is a powerful tool to understand the complexity of thrombosis initiation and provides both temporal and spatial resolution that cannot be obtained in vivo. The goal of this investigation is to develop a computational model of thrombosis initiation in patient-specific models that includes both a complex description of the hemodynamics and biochemistry of thrombin formation. We argue that the complex hemodynamics occurring in vivo significantly alter the initiation and progression of thrombosis.
While blood viscosity is known to exhibit nonlinear behavior, a Newtonian assumption is often employed in computational analyses. This assumption is valid in healthy arteries where shear rates are high and recirculation is low. However, in pathological geometries, such as aneurysms, and venous geometries, this assumption fails, and nonlinear viscous effects become exceedingly important. Previous computational models of thrombosis have investigated coagulation through chemistry based formulations focusing on protein dynamics but have generally excluded complex 3D hemodynamics.
A computational framework was developed to investigate the interplay between 3D hemodynamics and the biochemical reactions involved in thrombosis initiation in patient-specific models under transient flow. The salient features of the framework are: i) nonlinear rheological models of blood flow; ii) a stabilized numerical framework for scalar mass transport; and iii) a computational interface for nonlinear scalar models of protein dynamics that can be easily customized to include an arbitrary number of species and protein interactions.
We implemented and verified nonlinear rheological models of viscosity into CRIMSON and investigated the effects of non-Newtonian viscosity on both hemodynamic and transport metrics in an arterial and venous patient-specific model. Results demonstrated the importance of considering accurate rheological models.
A stabilized finite element (FE) framework was developed to solve scalar mass transport problems in CRIMSON. Simulation of cardiovascular scalar mass transport problems offers significant numerical challenges such as highly advective flows and flow reversal at outlet boundaries. Furthermore, little attention has been given to the identification of appropriate outflow boundary conditions that preserve the accuracy of the solution. These issues were resolved by developing a stabilized FE framework that incorporates backflow stabilization for Neumann outlet boundaries; a consistent flux boundary condition that minimally disturbs the local physics of the problem; and front-capturing stabilization to regularize solutions in high Pe number flows. The efficacy of these formulations was investigated for both idealized and patient-specific geometries.
Next, a flexible arbitrary reaction-advection-diffusion (ARAD) interface was implemented that enables prototyping nonlinear biochemical models of thrombin generation. After verifying the ARAD interface, the performance was compared against the original hardcoded FORTRAN implementation for speed and accuracy using a 4-scalar nonlinear reaction model of thrombosis. Three different biochemical models of thrombin generation were investigated in idealized geometries. Finally, we implemented the 18 scalar model in both idealized and patient-specific geometries to determine the effects of complex 3D hemodynamics on thrombin generation.
The computational framework for thrombosis initiation presented in this work has three key features: i) non-Newtonian hemodynamics; ii) a stabilized numerical framework for scalar RAD problems; and iii) a method to rapidly prototype custom reaction models using Python with negligible associated computational expense.
Chair: Prof. Alberto C. Figueroa
Explore Similar Events
-
Loading Similar Events...