Presented By: Biomedical Engineering
BME PH.D. Defense: Christopher D. Davidson
Biomaterial Control of Cell Forces to Enable Intercellular Communication During Vasculogenic Assembly
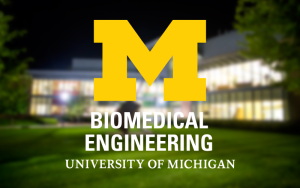
The ability of cells to communicate and coordinate their activity is crucial to the development and homeostatic function of all tissues. In addition to the well-established means of biochemically mediated signaling, a more recent body of evidence has indicated that cells can also communicate via cell-generated forces transmitted to neighboring cells through the extracellular matrix (ECM). One setting in which a deeper understanding of mechanical intercellular communication (MIC) would be extremely valuable is in vasculogenesis, or the de novo formation of a microvascular network. This dynamic process involves the assembly and organization of individual endothelial progenitor cells into an interconnected network of capillaries, thus requiring cellular communication and coordination over large spatial scales. If fully understood and harnessed, vasculogenic assembly presents a promising approach to vascularizing engineered tissue constructs for regenerative medicine applications. We hypothesize physical properties of the ECM are critical to MIC as the matrix context defines not only the generation of cell forces but also force transmission through the matrix to nearby cells. Thus, the focus of this dissertation is to study cell force propagation and MIC between endothelial cells (ECs) in controllable synthetic ECMs towards the informed design of biomaterials that drive rapid self-assembly of functional microvascular networks.
First, this thesis explores how physical attributes of the ECM regulate the assembly of ECs into interconnected multicellular networks. To mimic the fibrous microenvironments where neovascularization typically occurs in the body, we developed a novel model of the EC network formation assay utilizing 2.5D matrices of electrospun synthetic dextran methacrylate (DexMA) polymeric fibers. Our results revealed that active cell-mediated matrix deformations and fiber recruitment through actomyosin force generation occurs concurrently with the formation and stabilization of multicellular EC networks.
Next, this thesis describes the development and characterization of a new material system composed of electrospun dextran vinyl sulfone (DexVS) polymeric fibers that possess longer-term mechanical stability in culture as compared to DexMA matrices. These matrices were utilized for two major objectives: 1) investigating the role of matrix mechanics on the activation of fibroblasts into myofibroblasts, a key component of wound healing and the fibrotic progression, and 2) exploring the impact of nonlinear matrix mechanical properties on vasculogenic assembly by imbuing fibers with crimped microstructure.
Lastly, this thesis describes the mechanism of MIC between individual ECs during vasculogenic assembly. By combining electrospun DexMA fiber matrices with a microfabrication-based cell-patterning method, we investigated EC force-mediated matrix displacements and MIC as a function of matrix stiffness and identified the critical cellular machinery required for ECs to sense and respond to mechanical signals emanating from neighboring cells. We then sought to harness these observations in more translatable 3D hydrogel constructs by using a composite approach where fibrin hydrogels were reinforced with electrospun DexVS fiber segments. While traditional approaches to prevascularize 3D hydrogels require long-term co-cultures of ECs and support stromal cells, our work demonstrated that mechanical cues from synthetic fibers enable ECs alone to rapidly self-assemble into networks of lumenized capillary-like structures.
Overall, the work presented in this dissertation integrates biomaterials, tissue engineering, and microfabrication approaches to investigate the mechanobiology of how cell forces regulate intercellular communication during vasculogenic assembly. The results presented here are critical to the design of biomaterials that promote robust capillary network assembly for applications in tissue engineering and regenerative medicine.
Date: Friday, December 10, 2021
Time: 1:00 PM EST
Zoom: https://umich.zoom.us/meeting/register/tJAoceuhqD0rHdwL8duV6QwOcYjLXl14kysD (Zoom link requires prior registration)
Chair: Dr. Brendon Baker
First, this thesis explores how physical attributes of the ECM regulate the assembly of ECs into interconnected multicellular networks. To mimic the fibrous microenvironments where neovascularization typically occurs in the body, we developed a novel model of the EC network formation assay utilizing 2.5D matrices of electrospun synthetic dextran methacrylate (DexMA) polymeric fibers. Our results revealed that active cell-mediated matrix deformations and fiber recruitment through actomyosin force generation occurs concurrently with the formation and stabilization of multicellular EC networks.
Next, this thesis describes the development and characterization of a new material system composed of electrospun dextran vinyl sulfone (DexVS) polymeric fibers that possess longer-term mechanical stability in culture as compared to DexMA matrices. These matrices were utilized for two major objectives: 1) investigating the role of matrix mechanics on the activation of fibroblasts into myofibroblasts, a key component of wound healing and the fibrotic progression, and 2) exploring the impact of nonlinear matrix mechanical properties on vasculogenic assembly by imbuing fibers with crimped microstructure.
Lastly, this thesis describes the mechanism of MIC between individual ECs during vasculogenic assembly. By combining electrospun DexMA fiber matrices with a microfabrication-based cell-patterning method, we investigated EC force-mediated matrix displacements and MIC as a function of matrix stiffness and identified the critical cellular machinery required for ECs to sense and respond to mechanical signals emanating from neighboring cells. We then sought to harness these observations in more translatable 3D hydrogel constructs by using a composite approach where fibrin hydrogels were reinforced with electrospun DexVS fiber segments. While traditional approaches to prevascularize 3D hydrogels require long-term co-cultures of ECs and support stromal cells, our work demonstrated that mechanical cues from synthetic fibers enable ECs alone to rapidly self-assemble into networks of lumenized capillary-like structures.
Overall, the work presented in this dissertation integrates biomaterials, tissue engineering, and microfabrication approaches to investigate the mechanobiology of how cell forces regulate intercellular communication during vasculogenic assembly. The results presented here are critical to the design of biomaterials that promote robust capillary network assembly for applications in tissue engineering and regenerative medicine.
Date: Friday, December 10, 2021
Time: 1:00 PM EST
Zoom: https://umich.zoom.us/meeting/register/tJAoceuhqD0rHdwL8duV6QwOcYjLXl14kysD (Zoom link requires prior registration)
Chair: Dr. Brendon Baker
Explore Similar Events
-
Loading Similar Events...